Heat Stress and its Impact
Dr. Anthony E. Hall, Botany and Plant Sciences Department University of California, Riverside
The environmental and physiological nature of heat stress
Heat stress often is defined as where temperatures are hot enough for sufficient time that they cause irreversible damage to plant function or development. In addition, high temperatures can increase the rate of reproductive development, which shortens the time for photosynthesis to contribute to fruit or seed production. I also will consider this as a heat-stress effect even though it may not cause permanent (irreversible) damage to development because the acceleration does substantially reduce total fruit or grain yield.
The extent to which heat stress occurs in specific climatic zones is a complex issue. Plants can be damaged in different ways by either high day or high night temperatures and by either high air or high soil temperatures. Also, crop species and cultivars differ in their sensitivity to high temperatures. Cool-season annual species are more sensitive to hot weather than warm-season annuals. In Table 1 there are several examples of cool-season and warm-season annual crop species. I did not include safflower in the table because it is unusual in that during the vegetative stage it grows well in cool conditions and during the reproductive stage it grows well in hot conditions.
Table 1. Annual crop species adapted to cool and warm seasons (Hall 2001).
Cool-season annuals | Warm-season annuals |
Barley, brassicas, canola, fava bean, flax, garbanzo bean, Irish potato, lentil, lettuce, lupine, mustard, oat, pea, radish, rye, spinach, triticale, turnip, vetch, wheat | common bean, cotton, cowpea, cucurbits , finger millet, grain amaranth, lima bean, maize, mung bean, pearl millet, pepper, pigeon pea, rice sesame, sorghum, soybean, sunflower, sweet potato, tobacco, tomato |
High day temperatures can have direct damaging effects associated with hot tissue temperatures or indirect effects associated with the plant-water-deficits that can arise due to high evaporative demands. Evaporative demand exhibits near exponential increases with increases in day-time temperatures and can result in high transpiration rates and low plant water potentials (Hall 2001). The effects of drought and plant-water-deficits on crop adaptation are discussed in the ‘Drought Stress’ page.
Air temperatures vary during the day and season. Temperature data from weather stations for many locations in California may be found at the California Weather Databases linked to the Integrated Pest Management Project of the University of California. For many cities in the world, monthly means of daily maximum and minimum air temperatures may be found at the worldclimate web site. Additional world weather data may be found at the Global Climate Maps. Definitions of temperate, subtropical and tropical climatic zones and the average monthly means of daily maximum and minimum air temperatures that can occur in representative locations in these zones may be found in the book by Hall (2001).
The extent of heat stress that can occur in a specific climatic zone depends on the probability of high temperatures occurring and their duration during the day or night. Where global climate change is occurring these probabilities may not be predicted well based only on historical records for specific locations. Heat stress is a complex function of intensity (temperature degrees), duration and rate of increase in temperature. The magnitude of heat stress rapidly increases as temperature increases above a threshold level and complex acclimation effects can occur that depend on temperature and other environmental factors.
High soil temperatures can reduce plant emergence. The maximum threshold temperatures for germination and emergence are higher for warm-season than for cool-season annuals. For example, the threshold maximum seed zone temperature for emergence of cowpea is about 370C compared with 25 to 330C for lettuce.
During the vegetative stage, high day temperatures can cause damage to components of leaf photosynthesis, reducing carbon dioxide assimilation rates compared with environments having more optimal temperatures. Sensitivity of photosynthesis to heat mainly may be due to damage to components of photosystem II located in the thylakoid membranes of the chloroplast and membrane properties (Al-Khatib and Paulsen 1999). Membrane thermostability has been evaluated by measuring electrolyte leakage from leaf disks subjected to extreme temperatures (Blum 1988). More stable membranes exhibit slower electrolyte leakage. Studies comparing responses to heat of contrasting species indicated that photosystem II of the cool season species, wheat, is more sensitive to heat than photosystem II of rice and pearl millet, which are warm season species adapted to much higher temperatures (Al-Khatib and Paulsen 1999).
Extreme temperatures can cause premature death of plants. Among the cool-season annuals, pea is very sensitive to high day temperatures with death of the plant occurring when air temperatures exceed about 350C for sufficient duration, whereas barley is very heat tolerant, especially during grain filling. For warm season annuals, cowpea can produce substantial biomass when growing in one of the hottest crop production environments on earth (maximum day-time air temperatures in a weather station shelter of about 500C), although its vegetative development may exhibit abnormalities such as leaf fasciations. For monocotyledons, including both cool-season and warm-season annuals, high daytime temperatures can cause leaf firing which involves necrosis of the leaf tips and this symptom also can be caused by drought.
Reproductive development of many crop species is damaged by heat such that they produce no flowers or if they produce flowers they may set no fruit or seeds. The reviews of Hall (1992, 1993) discuss the detrimental effects of heat stress on reproductive development that has been reported for cowpea, common bean, tomato, cotton, rice, wheat, maize and sorghum. I will examine the detrimental effects of heat stress on cowpea because of the comprehensive information available for this species and the likelihood that many of the same phenomena occur with other warm-season crop species.
Controlled-environment studies in which cowpea plants were subjected to separately controlled root and shoot and day and night temperatures demonstrated that pod set (the proportion of flowers producing pods) was damaged by moderately high night temperature of the shoot (Warrag and Hall 1984a, b). It was surprising that night temperature would have this effect since much hotter day temperatures did not damage pod set of cowpea. Reciprocal artificial pollinations between plants grown under high and optimal night temperatures indicated the low pod set was caused by male sterility and that the pistils did not appear to be damaged by high night temperature. The detrimental effects of high night temperature on pod set also were shown to occur in field conditions (Nielsen and Hall 1985b). In these experiments a unique experimental approach was used in which plots of cowpea plants were subjected to different increments of higher night temperatures during early stages of flowering using enclosure systems placed over the plots only during the night-time (Nielsen and Hall 1985a).
Possible mechanisms for the sensitivity of pod set to high night temperatures have been proposed. Mutters and Hall (1992) demonstrated that there is a distinct period during the 24-hour cycle when pollen development in cowpea is sensitive to high night temperatures. Plants subjected to high temperature during the last six hours of the night exhibited substantially decreased pollen viability and pod set, whereas plants subjected to high temperature during the first six hours of a twelve-hour night exhibited no damage. Mutters and Hall (1992) hypothesized that these results could be explained if a heat-sensitive process in pollen development is under circadian control and only occurs in the late night period. Note that if a heat-sensitive process is under circadian control and if genetic variation exists for the time in the 24-hour cycle when this process occurs, evolution in hot environments would favor plants in which the heat-sensitive process occurs at the coolest time which is just prior to dawn. The damaging effect of high night temperature on pod set was greater in long days than in short days, and red and far red light treatments indicated it is a phytochrome-mediated response (Mutters et al. 1989b).
Studies were conducted in which cowpeas were transferred between growth chambers having high or optimal night temperatures (Warrag and Hall 1984b; Ahmed et al. 1992). They demonstrated that the stage of floral development most sensitive to high night temperature occurs 9 to 7 days prior to anthesis, which is after meiosis and coincides with release of pollen microspores from the tetrads. Damage due to high night temperature was associated with premature degeneration of the tapetal layer that provides nutrients to developing pollen, infertile pollen and in some genotypes anthers did not dehiscence. The transfer of proline from the tapetal layer to pollen was inhibited (Mutters et al. 1989a).
Comparisons of heat-sensitive and heat-tolerant cowpeas showed a genotypic association between sensitivity to heat during pod set and rapid leakage of electrolytes from leaf discs subjected to heat stress (Ismail and Hall 1999). Possibly, the damage to pollen development by high night temperatures may be in some way associated with a heat-induced malfunction in membrane properties.
Floral bud development also can be damaged by heat such that plants do not produce flowers. For cowpea, two weeks or more of consecutive or interrupted hot nights during the first month after germination caused complete suppression of floral bud development (Ahmed and Hall 1993). In extreme cases the floral buds become necrotic and die. In field conditions, the damage occurs under long days but not under short days. However, responses to red and far red light indicated the effect was only partially consistent with the system being mediated by phytochrome (Mutter et al. 1989b). The damaging effect of high night temperature and long days on floral bud development also depended on light quality whereas the damaging effect on pod set did not depend on light quality (Ahmed et al. 1993b). When growth chambers were used with relatively large amounts of fluorescent light and little incandescent light, such that the red/far red ratio was 1.9, floral buds were not suppressed in long-day high night temperature conditions, but pod set was very low. This artificial light system provides a useful experimental method for studying the effects of heat stress on pod set without complications due to heat stress effects on floral bud development. When growth chambers were used with lighting systems providing a red/far red ratio of 1.3 to 1.6, floral bud suppression was observed that was similar to what is obtained under long-day high night temperature conditions in the field where sunlight has a red/far red ratio of about 1.2.
There are two important conclusions from these studies. First, the use of growth chambers with lighting systems that mainly depend on fluorescent lights can result in either serious artifacts or methodological advantages when studying plant reproductive responses to heat stress. Second that intense shading of floral buds could reduce the red/far red ratio below 1.2 in field conditions and intensify the floral bud suppression effect. In densely sown fields of cowpea, individual plants that are suffering from competition and are tall and spindly can exhibit floral bud suppression even though night temperatures are not too hot.
Pods of different cowpea genotypes produce 9 to 20 ovules with many cultivars having 15 ovules, but pods rarely produce these many seeds per pod. Under optimal conditions two-thirds of the ovules may produce seed, whereas with high day or high night temperature (Warrag and Hall 1983) and other stresses, such as drought, fewer seeds are produced per pod. For most cultivars and stresses, it is the ovules at the blossom end of the pod that suffer embryo abortion and do not produce seed, resulting in the production of “pinched” pods.
Cowpea seeds produced under high day temperatures can have asymmetrical twisted cotyledons (Warrag and Hall 1984a). Germination of the seed is not influenced and this effect of heat stress may not be a major problem for commercial production. In contrast, heat-induced brown discoloration of cowpea seed coats can occur with some cultivars and be a major problem causing consumers to reject grain. Higher night temperatures resulted in progressively larger numbers of seed with larger areas of brown discoloration on seed coats (Nielsen and Hall 1985b).
The extent to which high-temperature damage to photosynthesis or reproductive development affect fruit or grain yield probably depends on the extent to which the photosynthetic source and the reproductive sink are limiting fruit or grain yield, and this may vary among species and cultivars.
Surface and internal tissues of tomato and citrus fruit can be damaged by the combination of high temperatures and intense solar radiation. High tissue temperatures also can damage cambium layers in exposed trunks and branches.
Repercussions of heat stress
The reduction in maximal emergence of annual crops due to hot soils can be so pronounced that yield of the economic product is reduced substantially. This can be a major problem for crops, such as lettuce, whose canopies are not very plastic and cannot compensate for the low emergence. Heat stress at emergence is a major problem for cool-season crops that are sown in the late summer in hot subtropical zones with the objective of producing a crop during the cooler weather in the fall. Warm-season crops also can experience this problem in those tropical zones and seasons where soil temperature can be extremely hot at sowing.
The acceleration of reproductive development by high temperatures may partially explain why the potential grain yields of warm season crops, such as rice and cowpea, usually are higher in the subtropics than the tropics. The extent of the acceleration of development of cowpea has been determined (Fig.1) by subjecting plants to different night temperatures in field conditions using temporary enclosures imposed only at night with regulated heating systems (Nielsen and Hall 1985a). Under the cool night temperatures of subtropical California (minimum night temperatures of 160C), individual cowpea pods took 21 days to proceed from anthesis to mature dry pod. In contrast, the pod development period of the same cowpea cultivar was only 14 days with the higher night temperatures that occur under tropical conditions (minimum night temperatures of 260C).

More rapid pod development may increase the extent of embryo abortion, and individual seed usually are smaller in tropical compared with subtropical conditions for the same cowpea cultivar. Cowpeas subjected to elevated night-temperature treatments produced smaller seed (Nielsen and Hall 1985b). The more rapid development of individual fruits also results in the overall reproductive period of the plant being shorter. Grain yields of cowpea cultivars grown with optimal management are much less in tropical zones than in subtropical zones mainly due to the shorter overall reproductive period caused by the hot nights of tropical zones (Hall et al. 1997). Acceleration of reproductive development also is a problem for cool season crops, such as wheat, growing in environments that are hot during reproductive development (reviewed by Hall 1992, 1993).
Direct evidence for heat-stress effects on grain yield was provided by the studies of Nielsen and Hall (1985b) in which cowpea was subjected to different increments of elevated night temperature under field conditions in a subtropical location in California (Fig.2).
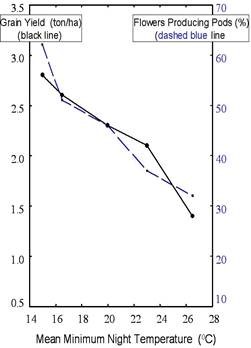
For minimum night temperatures greater than 150C there were linear reductions in both grain yield and the proportion of flowers that set pods with 50% reductions occurring at minimum night temperatures of about 260C.
Evaluating the same set of cultivars over a range of environments that mainly differ in temperature provides a more indirect approach for evaluating heat stress effects on the performance of crops that is of interest to farmers. Six heat-susceptible cowpea genotypes, including a California cultivar, were grown in eight field environments with contrasting temperatures but similar high levels of solar radiation and optimal management (Ismail and Hall 1998). Grain yield was negatively correlated with mean minimum night temperatures during the three-week period beginning one week before first flowering (Fig.3). For minimum night temperatures greater than 16.5oC grain yield decreased 13.6 % per oC associated with a similar decrease (11.5 % per oC) in number of pods per peduncle but only a small decrease (5.6 % per oC) in shoot biomass production.
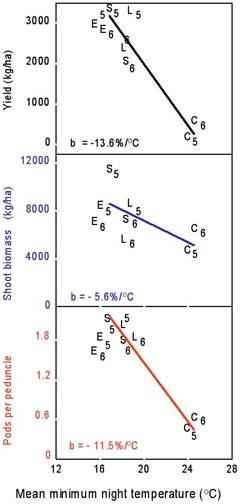
References
Ahmed, F. E. and A. E. Hall. 1993. Heat injury during early floral bud development in cowpea. Crop Sci. 33: 764-767.
Ahmed, F. E., A. E. Hall and D. A. DeMason. 1992. Heat injury during floral development in cowpea (Vigna Unguiculata, Fabaceae). Amer. J. Bot. 79:784-791.
Ahmed, F. E., A. E. Hall and M. A. Madore. 1993a. Interactive effects of high temperature and elevated carbon dioxide concentration on cowpea (Vigna unguiculata (L.) Walp.). Plant, Cell Environ. 16: 835-842.
Ahmed F. E., R. G. Mutters and A. E. Hall. 1993b. Interactive effects of high temperature and light quality on floral bud development in cowpea. Austral. J. Plant Physiol. 20: 661-667.
Al-Khatib, K. and G. M. Paulsen. 1999. High-temperature effects on photosynthetic processes in temperate and tropical cereals. Crop Sci. 39: 119-125.
Blum, A. 1988. Plant Breeding for Stress Environments. CRC Press, Inc., Boca Raton, Florida, pp 223.
Blum, A., N. Klueva and H. T. Nguyen. 2001. Wheat cellular thermotolerance is related to yield under heat stress. Euphytica 117: 117-123.
Cornish, K., J. W. Radin, E. L. Turcotte, Z. Lu and E. Zeiger. 1991. Enhanced photosynthesis and stomatal conductance of pima cotton (Gossypium barbadense L.) bred for increased yield. Plant Physiol. 97: 484-489.
Ehlers, J. D. and A. E. Hall. 1996. Genotypic classification of cowpea based on responses to heat and photoperiod. Crop Sci. 36: 673-679.
Ehlers, J. D. and A. E. Hall. 1998. Heat tolerance of contrasting cowpea lines in short and long days. Field Crops Res. 55: 11-21.
Ehlers, J. D., A. E. Hall, P. N. Patel, P. A. Roberts and W.C. Matthews. 2000. Registration of ‘California Blackeye 27′ cowpea. Crop Sci. 40: 854-855.
Fischer, R. A. 1985. Number of kernels in wheat crops and the influence of solar radiation and temperature. J. Agric. Sci. Camb. 105: 447-461.
Fischer, R. A. and R. Maurer. 1978. Drought resistance in spring wheat cultivars. I. Grain yield responses. Austr. J. Agric. Res. 29: 897-912.
Hall, A. E. 1992. Breeding for heat tolerance. Plant Breed. Rev. 10: 129-168.
Hall, A. E. 1993. Physiology and breeding for heat tolerance in cowpea, and comparison with other crops. Pp. 271-284, in C. G. Kuo (ed.) Adaptation of Food Crops to Temperature and Water Stress, Publ. No. 93-410, Asian Vegetable Research and Development Center, Shanhua, Taiwan.
Hall, A. E. 2001. Crop Responses to Environment. CRC Press LLC, Boca Raton, Florida.
Hall, A. E. and L. H. Ziska. 2000. Crop breeding strategies for the 21st century. Pp. 407-423 in K. R. Reddy and H. F. Hodges (eds.) Climate Change and Global Crop Productivity, CABI Publishing, New York, USA.
Hall, A. E., B. B. Singh and J. D. Ehlers. 1997. Cowpea breeding. Plant Breed. Rev. 15: 215-274.
Ismail, A. M. and A. E. Hall. 1998. Positive and negative effects of heat-tolerance genes in cowpea. Crop Sci. 38: 381-390.
Ismail, A. M. and A. E. Hall. 1999. Reproductive-stage heat tolerance, leaf membrane thermostability and plant morphology in cowpea. Crop Sci. 39: 1762-1768.
Ismail, A. M. and A. E. Hall. 2000. Semidwarf and standard-height cowpea responses to row spacing in different environments. Crop Sci. 40: 1618-1623.
Ismail, A. M., A. E. Hall and T. J. Close. 1997. Chilling tolerance during emergence of cowpea associated with a dehydrin and slow electrolyte leakage. Crop Sci. 37: 1270-1277.
Ismail, A. M., A. E. Hall and T. J. Close. 1999. Allelic variation of a dehydrin gene cosegregates with chilling tolerance during seedling emergence. PNAS 96: 13566-13570.
Jefferson, R. A. 1993. Beyond model systems – new strategies, methods and mechanisms for agricultural research. Ann. New York Acad. Sci. 700: 53-73.
Lee, G. J. and E. Vierling. 2000. A small heat shock protein cooperates with heat shock protein 70 systems to reactivate a heat-denatured protein. Plant Physiol. 122: 189-197.
Lu, Z., R. G. Pearcy, C. O. Qualset and E. Zeiger. 1998. Stomatal conductance predicts yields in irrigated Pima cotton and bread wheat grown at high temperatures. J. Exp. Bot. 49: 453-460.
Lu, Z., J. W. Radin, E. L. Turcotte, R. Percy and E. Zeiger. 1994. High yields in advanced lines of Pima cotton are associated with higher stomatal conductance, reduced leaf area and lower leaf temperature. Physiol. Plant. 92: 266-272.
Marfo, K. O. and A. E. Hall. 1992. Inheritance of heat tolerance during pod set in cowpea. Crop Sci. 32: 912-918.
Murakami, Y., Tsuyama, M., Kobayashi, Y., Kodama, H. and K. Iba. 2000. Trienoic fatty acids and plant tolerance of high temperature. Science 287: 476-479.
Mutters, R. G. and A. E. Hall. 1992. Reproductive responses of cowpea to high temperature during different night periods. Crop Sci. 32: 202-206.
Mutters, R. G., L. G. R. Ferreira and A. E. Hall. 1989a. Proline content of the anthers and pollen of heat-tolerant and heat-sensitive cowpea subjected to different temperatures. Crop Sci. 29: 1497-1500.
Mutters, R. G., A. E. Hall and P. N. Patel. 1989b. Photoperiod and light quality effects on cowpea floral development at high temperatures. Crop Sci. 29: 1501-1505.
Nielsen, C. L. and A. E. Hall. 1985a. Responses of cowpea (Vigna unguiculata [L.] Walp.) in the field to high night temperature during flowering. I. Thermal regimes of production regions and field experimental system. Field Crops Res. 10: 167-179.
Nielsen, C. L. and A. E. Hall. 1985b. Responses of cowpea (Vigna unguiculata [L.] Walp.) in the field to high night temperatures during flowering. II. Plant responses. Field Crop Res.10: 181-196.
Patel, P. N. and A. E. Hall. 1988. Inheritance of heat-induced brown discoloration in seed coats of cowpea. Crop Sci. 28: 929-932.
Reynolds, M. P. and E. E. Ewing. 1989. Effects of high air and soil temperature stress on growth and tuberization in Solanum tuberosum. Annals Bot. 64: 241-247.
Reynolds, M. P., M. Balota, M. I. B. Delgado, I. Amani and R. A. Fischer. 1994. Physiological and morphological traits associated with spring wheat yield under hot, irrigated conditions. Austral. J. Plant Physiol. 21: 717-730.
Saadala, M. M., J. S. Quick and J. F. Shanahan. 1990a. Heat tolerance in winter wheat. II. Membrane thermostability and field performance. Crop Sci. 30: 1248-1252.
Saadala, M. M., J. F. Shanahan and J. S. Quick. 1990b. Heat tolerance in winter wheat. I. Hardening and genetic effects on membrane thermostability. Crop Sci. 30: 1243-1247.
Shanahan, J. F., I. B. Edwards, J. S. Quick and J. R. Fenwick. 1990. Membrane thermostability and heat tolerance of spring wheat. Crop Sci. 30: 247-251.
Shpiler, L. and A. Blum. 1991. Heat tolerance for yield and its components in different wheat cultivars. Euphytica 51: 257-263.
Soman, P. and J. M. Peacock. 1985. A laboratory technique to screen seedling emergence of sorghum and pearl millet at high soil temperature. Expl. Agric. 21: 335-341.
Thiaw, S. 2003. Association between slow leaf-electrolyte-leakage under heat stress and heat tolerance during reproductive development in cowpea. Ph.D. Dissertation, University of California, Riverside.
Vierling, E. 1991. The roles of heat shock proteins in plants. Ann. Rev. Plant Physiology Plant Mol. Biol. 42: 579-620.
Warrag, M. O. A. and A. E. Hall. 1983. Reproductive responses of cowpea to heat stress: genotypic differences in tolerance to heat at flowering. Crop Sci. 23: 1088-1092.
Warrag, M. O. A. and A. E. Hall. 1984a. Reproductive responses of cowpea [Vigna unguiculata (L.) Walp.] to heat stress. I. Responses to soil and day air temperatures. Field Crops Res. 8: 3-16.
Warrag, M. O. A. and A. E. Hall. 1984b. Reproductive responses of cowpea [Vigna unguiculata (L.) Walp.] to heat stress. II. Responses to night air temperatures. Field Crops Res. 8: 17-33.